Decommissioned and abandoned mines are being turned into long-term energy storage solutions through methods such as pumped storage hydropower and underground gravity energy storage. Martin Morris speaks with Julian Hunt, research scholar, Energy, Climate and Environment Program, International Institute for Applied Systems Analysis; Rebecca Ellis, energy policy manager, International Hydropower Association; and Mark Swinnerton, founder and CEO of Green Gravity, to discuss the advantages and challenges of the various methods in play – and the benefits they can offer society at large.
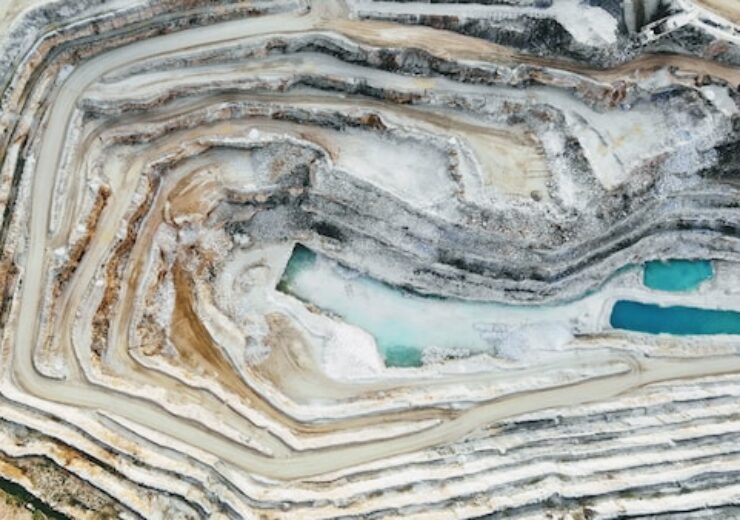
Unlocking the potential of abandoned mines for long-term energy storage. (Credit: Dion Beetson on Unsplash)
According to the US Department of Energy, pumped storage hydropower (PSH) accounted for 93% of all utility-scale energy storage in the US in 2021.
A form of hydroelectric energy storage, PSH is based on a configuration of two water reservoirs at different elevations, generating power as water moves down from one to the other – known as ‘discharge’ – while passing through a turbine. When the opposite holds true and water is being pumped back into the upper reservoir during the recharge phase, power will be required to complete the process.
As a result, PSH can be viewed as tantamount to a giant battery, given it can store power and then release it when required. PSH can also be characterised as ‘open-loop’ or ‘closed-loop’. Open-loop PSH has an ongoing hydrologic connection to a natural body of water, while in the case of closed-loop PSH, reservoirs aren’t connected to any outside body of water.
On the face of it, open-cycle hydropower plants are cheaper, because they can take advantage of the available natural topography and geology – only requiring a large upper reservoir and a smaller lower one connected to a river. Yet, as Julian Hunt, of the International Institute for Applied Systems Analysis (IIASA), is quick to point out, much will be determined by river flow and overall demand for the water. One potential water conflict resulting from open-cycle seasonal pumped hydropower storage plants (SPHS), for example, is that most water is consumed during the summer – invariably for agricultural purposes. However, the SPHS would store water during the summer and release it during the winter when water demand is low. Closed-cycle plants, on the other hand, are more expensive, because they require large upper and lower reservoirs. “Their main benefit is that water is reused in the storage cycle and water conflicts are significantly reduced,” says Hunt.
Scoping potential new sites
Meanwhile, Rebecca Ellis, energy policy manager at the International Hydropower Association (IHA), citing the Australian National University (ANU)’s Global PSH Atlas, notes there are currently an estimated 616,000 potentially feasible off-river PSH sites globally with storage potential of about 23m gigawatt-hours.
“In addition to potential new sites,” says Ellis, “existing plant modernisation can prolong the life of the assets and increase the range of flexibility services that hydropower assets can provide to the electricity system.”
“IHA are currently supporting the XFLEX Hydropower project, an EU Horizon 2020 project,” she adds. “This is a demonstration project to showcase the means in which hydropower and PSH assets can provide a range of invaluable grid services to ensure that local and regional power grids remain reliable and resilient to current and future disruptions.”
Hydropower provides very low-cost electricity over its long lifetime, despite the relatively high construction costs upfront. The big questions, however, are over whether costs will continue to come down as a result of technological advances and whether business use cases can be made. For Ellis, there’s a simple answer. “Based on levelised cost of electricity (LCOE) analysis by the International Renewable Energy Agency (IRENA) from 2022, hydropower is regarded as the second-cheapest renewable energy. This is especially important when considering the long lifetime of the assets – both machinery and civil works.”
However, Ellis also sounds a note of caution. “Because of the large upfront costs, investors are exposed to a significant proportion of the lifetime cost of the project before revenues commence,” she says. However, public sector financial measures to support projects, and international good practices like the Hydropower Sustainability Standard, can be used to help mitigate risk for investors.
“Lack of remuneration for the essential grid services that PSH and hydropower currently provides – and will provide in the future – is something that national governments need to address in order to help hydropower projects,” Ellis reiterates. However, “electricity markets, especially during the context of the energy transition, are immensely difficult to predict, meaning there is a lack of predictable revenue streams over the long period of project development; thereby making it difficult for developers”.
Gravity check
While PSH is firmly established, it is by no means the only game in town when it comes to energy storage solutions, particularly when viewed in the longer term – the case in point being the emergence of underground gravity energy storage (UGES). Where safe and technically feasible, there is significant potential to repurpose previously operational mines – one example being Dinorwig, a repurposed slate mining facility in northern Wales that operating since 1984, which provides a 1,728MW PSH, according to Ellis. Another example is in Estonia, where Festi Energia is in the planning phase of repurposing a mine in Ida-Virumaa County, using the limestone rubble and closed tunnels previously deployed for mining oil shale. Ultimately, it’s expected to have a capacity of 225MW and is slated to begin operations in 2026.
Noteworthy too is the Kidston project in Australia, which is currently in stage two of development and is the first energy storage project that will make use of an abandoned gold mine. It’s projected to produce 250MW and will incorporate solar PV. In the meantime, the UGES model proposed by IIASA researchers, for example, uses existing elevators to raise and lower containers full of sand. Mines are well-suited to such batteries – principally because they already have deep shafts that can be used to drop a weight. UGES generates electricity when the price is high by lowering sand into an underground mine and converting the potential energy of the sand into electricity via regenerative braking and then lifting the sand from the mine to an upper reservoir using electric motors to store energy when electricity is cheap.
The main components of UGES are the shaft, motor and generator, upper and lower storage sites, and mining equipment. The deeper and broader the mineshaft, the more power can be extracted from the plant, and the larger the mine, the higher the plant’s energy storage capacity, according to IIASA.
Energy storage in the long-term
The key takeaway here, however, is that while energy storage methods – such as batteries – lose energy via self-discharge over long periods; using sand enables ultra-long time energy storage ranging from weeks to even several years. As IIASA’s Julian Hunt points out, “Comparing SPHS and UGES, there are several SPHS plants worldwide, particularly in Austria, Switzerland, Italy, France, Norway, USA and Japan.
“SPHS is a better option [over] UGES because power – tunnels, turbines, generators – and energy storage – dam, water, land – costs are low. This makes SPHS an option to store energy in hourly, daily, weekly, monthly, seasonal and pluriannual cycles with the same plant. However, much will depend on the topography and water availability. SPHS would only be able to supply some of the long-term energy demands of Europe, for example, because of a lack of water and a possible conflict over water use.”
In Australia, meanwhile, Green Gravity’s energy storage solution offers a practical guide to energy storage – harnessing the principles of gravity and kinetic energy to store and dispatch energy by lifting and lowering heavy-weighted objects.
Due to basic physics, the amount of energy created will be less than the amount of energy used to lift the heavy objects to the surface. Indeed, this is the case for all energy storage devices – batteries, pumped hydro and so on – as there is always some loss of energy as it is converted between forms, according to Green Gravity Founder and CEO, Mark Swinnerton.
“Energy storage technologies can see efficiency levels of 50–90% depending on their nature,” says Swinnerton. “Because of these energy losses, it is preferable to use energy directly rather than store it. However, as we increase renewable production it becomes more difficult to directly consume all of the production, necessitating the use of energy storage.”
Gravity remains key to storage
Swinnerton notes that gravity energy storage systems deliver around 80% energy efficiency. “For our technology, the energy losses are caused by things like heat in motors, friction from ropes and the use of energy to manage the logistics of the heavy objects.”
So, the obvious question is, how can gravity energy storage systems make economic sense, given the associated energy losses – as with other methods? For Swinnerton, the answer needs to be viewed through the prism of supply-and-demand-driven pricing in the wholesale power markets.
“When there is excess renewable energy production – say at lunchtime – the power price is low, and when there is high demand and low renewable production – say, at dinnertime – the price is high. If an energy storage device is charged when the price is low and discharged when the price is high, then the economics can work,” says Swinnerton. Self-evidently, reducing energy inefficiency and loss is always the objective. Unsurprisingly, for Green Gravity, addressing such issues is an ongoing process technologically.
“The most important technical challenge for Green Gravity is how to install the energy storage system in a wide variety of legacy mine sites. Researching how to adapt the system to a wider set of conditions will increase the number of locations our technology can be installed, thereby increasing the benefits to the renewable energy transition,” Swinnerton says.
“The supply chain benefits of gravitational energy storage technologies is significant,” he adds. “Green Gravity is able to repurpose mines and, along with that, a portion of infrastructure. The components utilised in our system are proven and available, and in most cases are available locally in countries. Some other storage technologies, particularly chemical storage systems, utilise strategic or rare resources in their supply chains.”
Any policy vacuum needs to be filled
Naturally, given Green Gravity is based in Australia, Swinnerton needs to keep a constant eye on Australian government policy in this particular space. And while the government there has been “getting it right” in some areas – such as with the establishment of the Australian Renewable Energy Authority (ARENA) and the Clean Energy Finance Corporation (CEFC), improvements are needed elsewhere. While there are well-established processes and signals to bring energy storage to the market, more can be done to set clearer price signals for the future needs of the grid and to incentivise a wider set of metrics to be required in the provision of capacity – such as sovereign capacity, sustainability and so on – according to Swinnerton.
Specifically, for storage assets in the National Electricity Market (NEM), the fee structures – such as the Distribution Use of System (DUoS) – are outdated and not well suited for renewable energy storage, which is creating a disincentive to bring technology to market, says Swinnerton. His experience in Australia, however, confirms a wider truth in the gravity energy storage space – namely, that technological advances will likely be less relevant unless local government policies and initiatives are in place to underpin them as well. That would then make the business use case easier for would-be participants.
This article first appeared in World Mining Frontiers magazine.